Ashley Liaw
College of Human Ecology
Department of Human Centered Design Cornell University
21 December 2022
Green Electrospinning of Controlled-release Quercetin/Cyclodextrin Inclusion Complex Nanofibrous Webs for Medical Applications
1. INTRODUCTION
Within the biomedical textile industry, a highly significant sub sector is wound dressings, valued at 13.7 billion USD in 2022 (1). However, among surface wound applications, there are few products that allow for time-regulated release of medicinal chemicals on damaged skin regions. Most products require additional application of creams or medicines which quickly dissolve into the skin, providing a one time treatment.
Quercetin/cyclodextrin inclusion complex nanofibrous webs resolve this issue by allowing for the moderated-release of quercetin to provide oxidative and anti-bacterial properties for wound healing. The objective of this study is to analyze and perform synthesis of Quercetin/Hydroxypropyl-beta-Cyclodextrin (HP-β-CyD) and Quercetin/Hydroxypropyl-gamma-Cyclodextrin (HP-γ-CyD) inclusion complex nanofibrous webs through electrospinning for the controlled-release of quercetin.
Electrospinning is a nanofiber formation technique which utilizes high voltages to eject liquid solution jets, ultimately providing elongation into fibers (2). This process dates back to 1600 when the concept was first illustrated by William Gilbert all the way to 1938 when the first electrospun nanofibers were used in the Soviet Union for air filters (2). Advancement appeared in the 1990s through use of a variety of new materials in the spinning process and increased ability to modify structure and alignment of resultant nanofibers (2). Within time-regulated drug release with electrospun nanofibers, there have been much literature involving wound dressings incorporating various antibiotic, antibacterial, and antifungal drugs (2), but there has been smaller exploration with controlled release of drugs, specifically quercetin.
Quercetin is a bioactive flavonoid which provides antioxidant properties, protecting cells from unstable molecules and cell tissues from toxic drugs (3). Additionally, the bioflavonoid is antiviral, anti-allergic, anti-inflammatory, and protects against free radicals which are a main cause of many diseases such as hypertension and metabolic syndrome (3). Quercetin is found in many plant sources such as berries, onions, broccoli, and citrus fruits (3). Thus, quercetin’s positive health and recovery properties, in addition to its green sourcing, make it a suitable drug to achieve increased wound healing in skin injury dressings.
2. MATERIALS AND METHODS
2.1 Materials
Hydroxypropyl-beta-cyclodextrin (HP-β-CyD) (Cavasol W7 HP, DS: ~0.9) and Hydroxypropyl-gamma-cyclodextrin (HP-γ-CyD) (Cavasol W8 HP Pharma, DS: ~ 0.6) were sourced from Wacker Chemie AG (USA). Sodium hypophosphite hydrate (NaPO2H2, Sigma-Aldrich), Citric acid ( >99.5%, Alfa Aesar), and Quercetin hydrate (95%, Thermoscientific) were purchased and used without further purification. Distilled water was used from the Millipore Milli-Q ultrapure water system (Millipore, USA).
2.2 Preparation of Solutions for Electrospinning
Various nanofibrous films were electrospun from solutions with several different ratios of Quercetin to HP-β-CyD or HP-γ-CyD. Specifically, solutions with molar ratios of 1:1, 1:2, 1:4, and 1:8 of Qu:CyD were created and electrospun.
Designated masses of HP-β-CyD or HP-γ-CyD were measured and added to a drum vial followed by 500 microliters of distilled water. Vials were stirred on a hot plate for around 20 minutes until reaching a homogenous solution followed by addition of designated masses of quercetin. After drug addition, solutions were left to stir for 24 hours for formation of inclusion complexes.
Some of the nanofibrous films went through the additional step of crosslinking through inclusion of citric acid and sodium hypophosphite hydrate to the solution after the 24 hour stirring period. Crosslinked solutions required 10-15 minutes of additional stirring followed by cooling to room temperature. Cooled solutions were placed in 1 mL syringes, attached to 27 Gauge needles.
Syringes were mounted into a syringe pump within the electrospinning instrument. A collector plate prepared with aluminum foil and a glass slide was also placed opposite from the mounted syringe. Climate controls were set followed by voltage levels (17,500 eV) and ejection speeds (0.5 ml/hr).
Nanofibers then began to form on the collector plate. Once an evenly distributed layer of nanofibers accumulated, the glass slide was removed and examined under an optical microscope to examine the nanofiber morphology and electrospinning progress.
2.3 Solution Properties
Properties of each solution created were examined through conductivity and viscosity tests. Conductivity was determined using a conductivity-meter at room temperature. Three conductivity values were recorded for each sample. Viscosity of each sample was determined through use of a rheometer.
2.4 Morphological and Composition Analysis
Further morphological analysis was conducted through Scanning Electron Microscopy (SEM) where small portions of the nanofiber samples were cut and attached to metal stubs with conductive tape. Stubs were then placed within the scanning electron microscope without sputter coating. Working distance was set to around 10 mm while accelerating voltage was 12.0 kV.
Fourier Transform Infrared Spectroscopy (FTIR) was also utilized to analyze the nanofibrous web composition. Specifically, small amounts of each sample were placed onto a sample plate and compressed for analysis.
2.5 Thermocharactization and Structural Analysis
Differential Scanning Calorimetry (DSC) was performed to identify sample thermal properties, especially in comparing HP-β-CyD and HP-γ-CyD sample properties. Heat flow versus temperature allows for one to examine sample components such as drug encapsulation due to phase change and melting point data. However, the differential scanning calorimeter used was unable to surpass 300°C near melting points of HP-β-CyD and HP-γ-CyD. Thus, DSC was not effective in comparing the two types of cyclodextrins.
Instead, X-ray Diffraction (XRD) through use of an x-ray diffractometer aided in HP-β-CyD and HP-γ-CyD comparison through analyzing sample crystallinity and structure.
Thermogravimetric Analysis (TGA) was used to perform further thermal analysis on samples by analyzing weight change corresponding to increasing sample temperature heating. A thermogravimetric analyzer was used, heated from room temperature to 600°C.
2.6 Sustained Release Test
Release rates of quercetin within the Qu/CyD nanofibrous webs was determined through immersing tiny portions of nanofibrous samples in 7.4 pH and 5.4 pH buffer solutions followed by placement on a shaker instrument at 33°C at a speed of 150 rpm. Simultaneously, UV-Vis spectroscopy of samples was conducted after various time intervals ranging up to 8 hours. 7.4 pH and 5.4 pH buffer were utilized to replicate the pH levels of unwounded and wounded skin.
2.7 Antioxidant Activity Test
A 2,2-diphenyl-1-picrylhydrazyl (DPPH) solution was made to examine the antioxidant activity of each sample. The DPPH solution was created by dissolving in methanol. A small portion of the nanofibrous web was cut and incubated into the solution for various time intervals followed by UV–Vis spectroscopy, allowing for determination of solution absorbance.
3. RESULTS AND DISCUSSION
3.1 Morphology of Nanofibrous Films
During the electrospinning process, glass slides were placed on collectors to analyze periodic nanofiber formation under a microscope. The microscope images and glass slides for four samples may be seen in Figure 1. At the electrospinning midpoint where slides were examined, Figure 1 illustrates that all samples had some successful formation of nanofibers with samples (c) and (d) reaching higher levels of nanofibrous web formation.
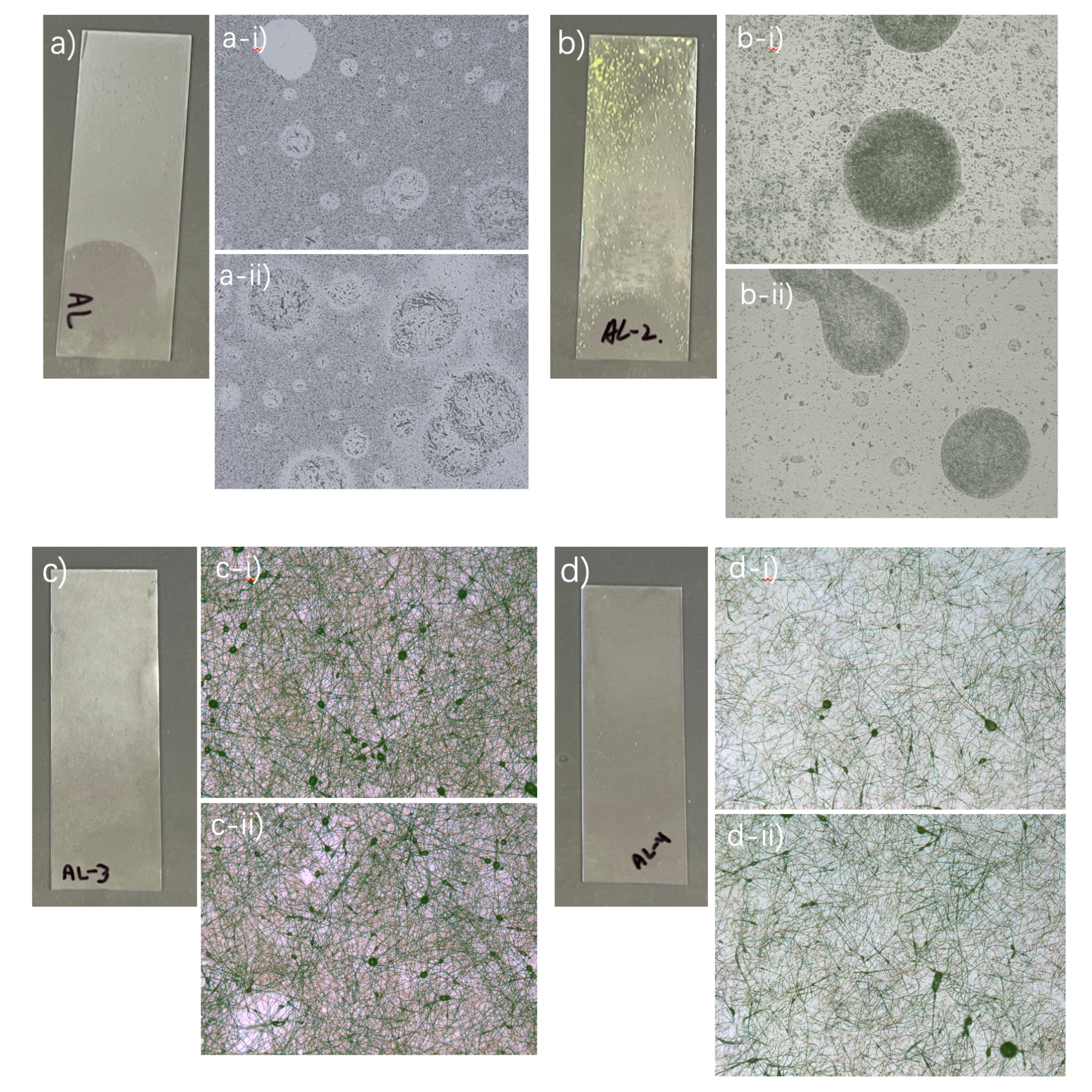
Figure 1. Fiber Formation and Structure Through Optical Microscopy of Samples (a) 180% Qu/β-CD (1:8), (b) 160% Qu/ɣ-CD (1:8), (c) 150% Qu/β-CD (1:8), and (d) 180% Qu/ɣ-CD (1:8)
Post-electrospun samples were further examined through SEM in order to determine nanofiber morphology. Figure 2 illustrates SEM imaging of four Qu-CyD samples. From this data, it can be noted that HP-β-CyD nanofibrous webs resulted in a smaller nanofiber diameter compared to HP-ɣ-CyD nanofibers. Additionally, nanofibrous webs with 1:4 concentration of Qu:CyD illustrated more beading compared to 1:8 concentration ratios. 1:8 nanofibers were longer and shown to have a more homogenous structure. In comparison of HP-β-CyD and HP-ɣ-CyD, HP-β-CyD demonstrated less beading, allowing it to have higher fiber quality than HP-ɣ-CyD.
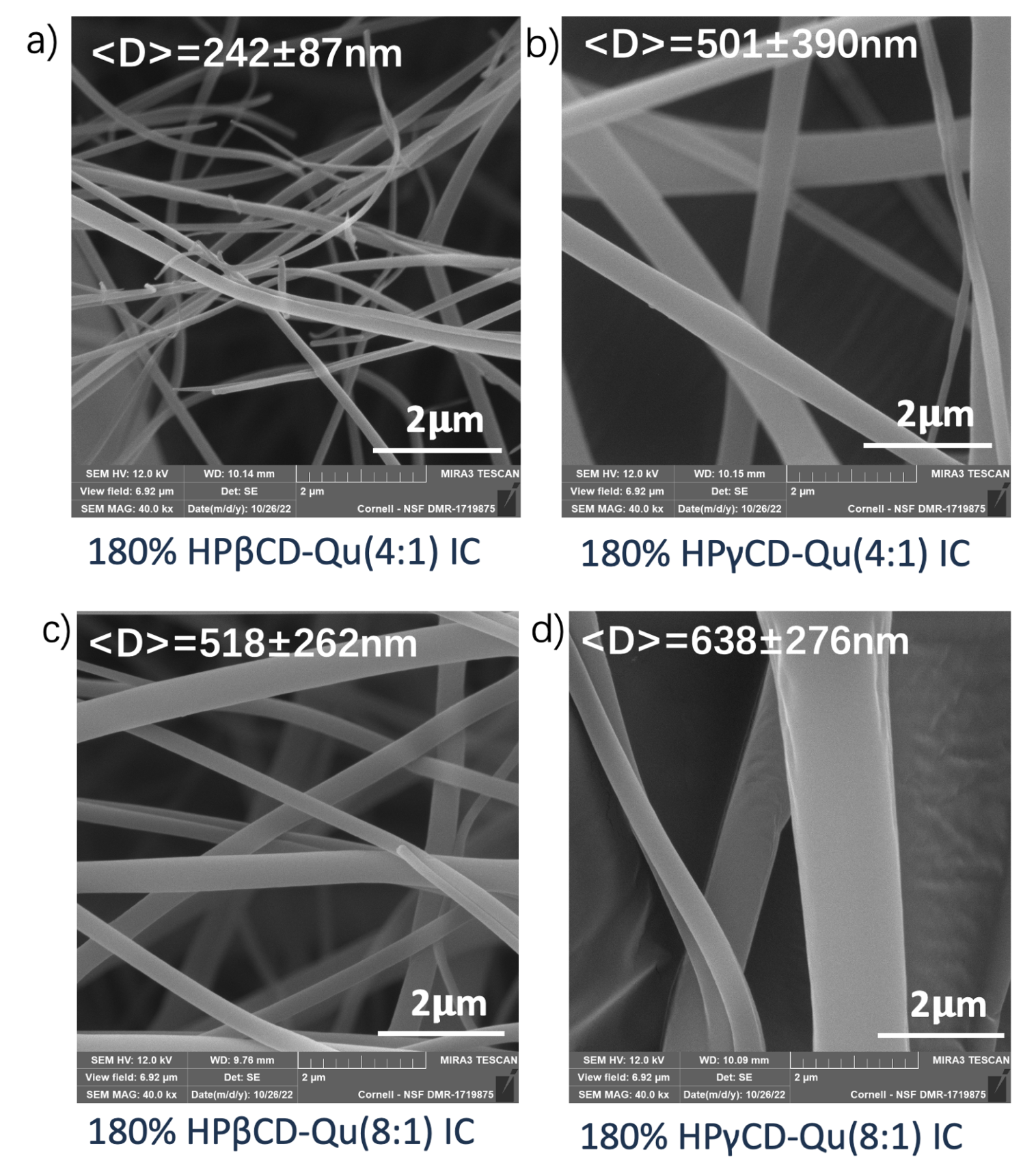
Figure 2. SEM Analysis of (a) 180% Qu/β-CD (1:4), (b) 180% Qu/ɣ-CD (1:4), (c) 180% Qu/β-CD (1:8), and (d) 180% Qu/ɣ-CD (1:8) (Credit to Ruobai Xiao)
3.2 Viscosity and Conductivity Analysis
Similar to the conclusion drawn from SEM analysis of the samples, HP-β-CyD nanofibrous webs had smaller nanofiber diameters compared to HP-ɣ-CyD nanofibers as illustrated by the viscosity test. Specifically, Table 1 illustrates how HP-ɣ-CyD nanofibers had higher viscosity values than their HP-β-CyD counterparts, corresponding to a thicker diameter.
The conductivity test illustrated how HP-ɣ-CyD-based nanofibrous webs had higher conductivity values, meaning these samples had higher concentrations of cyclodextrin and quercetin. Crosslinking fibers allowed for more conductivity and drug encapsulation. However, solutions containing the crosslinking agents of SHP and citric acid faced more difficulty in the electrospinning process. Thus, these samples were not able to be further examined.
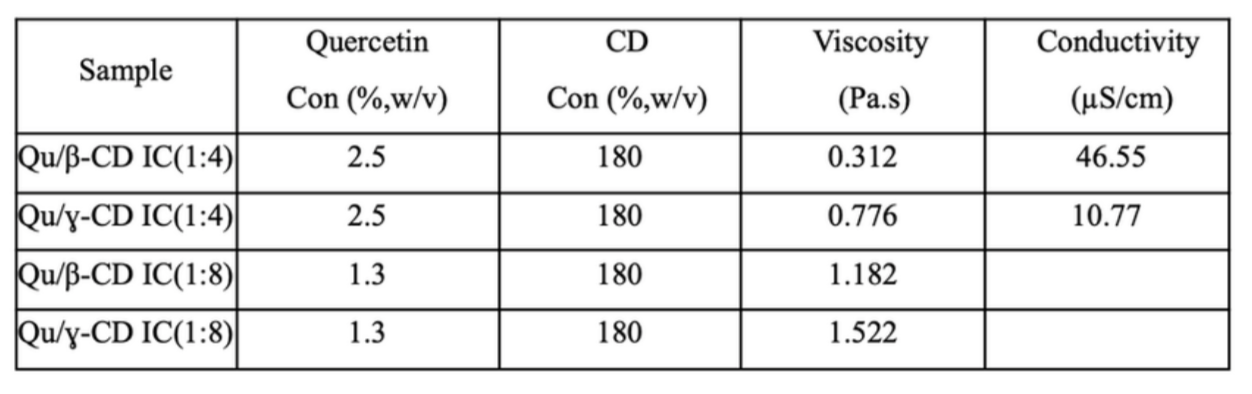
Table 1. Conductivity and Viscosity Analysis of Qu/β-CD IC(1:4), Qu/ɣ-CD IC(1:4), Qu/β-CD IC(1:8), and Qu/ɣ-CD IC(1:8)
3.3 Thermal Behavior Analysis
DSC analysis was conducted to examine the heat flow of each sample, as illustrated by the thermograms in Figure 3. As aforementioned, the differential scanning calorimeter used was unable to reach temperatures near melting points of HP-β-CyD and HP-γ-CyD, preventing DSC from being effective in comparing HP-β-CyD and HP-γ-CyD.
Within Figure 3a and 3b, similar peaks of individual HP-β-CyD, HP-γ-CyD, and quercetin may be seen within the combined Qu/CyD curves, reinforcing the successful encapsulation and presences of quercetin within nanofibrous webs.
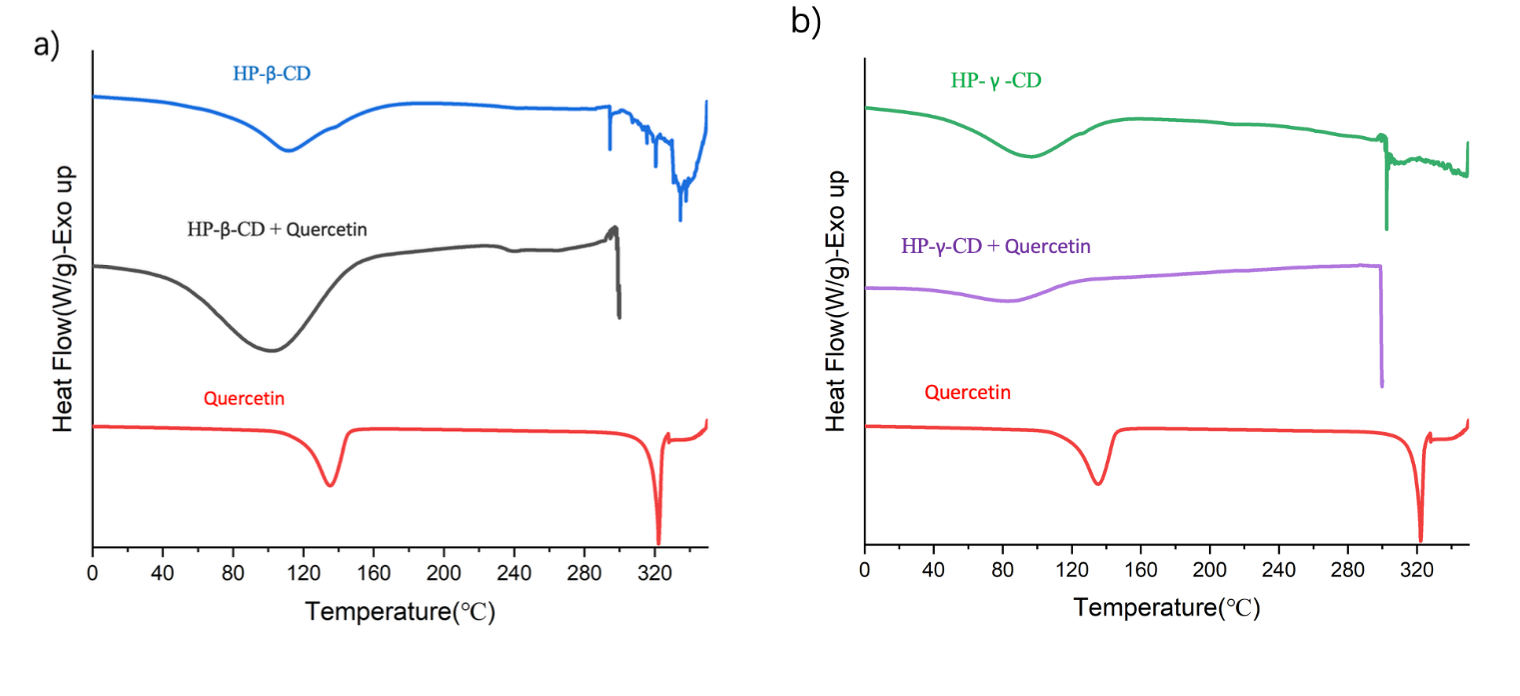
Figure 3. Differential Scanning Calorimetry of Quercetin + HP-β-CyD and Quercetin + HP-γ-CyD (Credit to Ruobai Xiao)
TGA was performed for further thermal analysis. Figure 4 illustrates how there were similar weight change inflection points for HP-β-CyD and HP-γ-CyD samples with and without quercetin. This inflection point occurs approximately around 350°C which is close to that of quercetin illustrated in the graph. The similar inflection points demonstrate fairly high purity and desired composition of drug-encapsulated samples.
In terms of FTIR analysis, there was not enough time to determine this data for all samples. However, an adequate understanding of the process was attained through shadowing execution of the process.
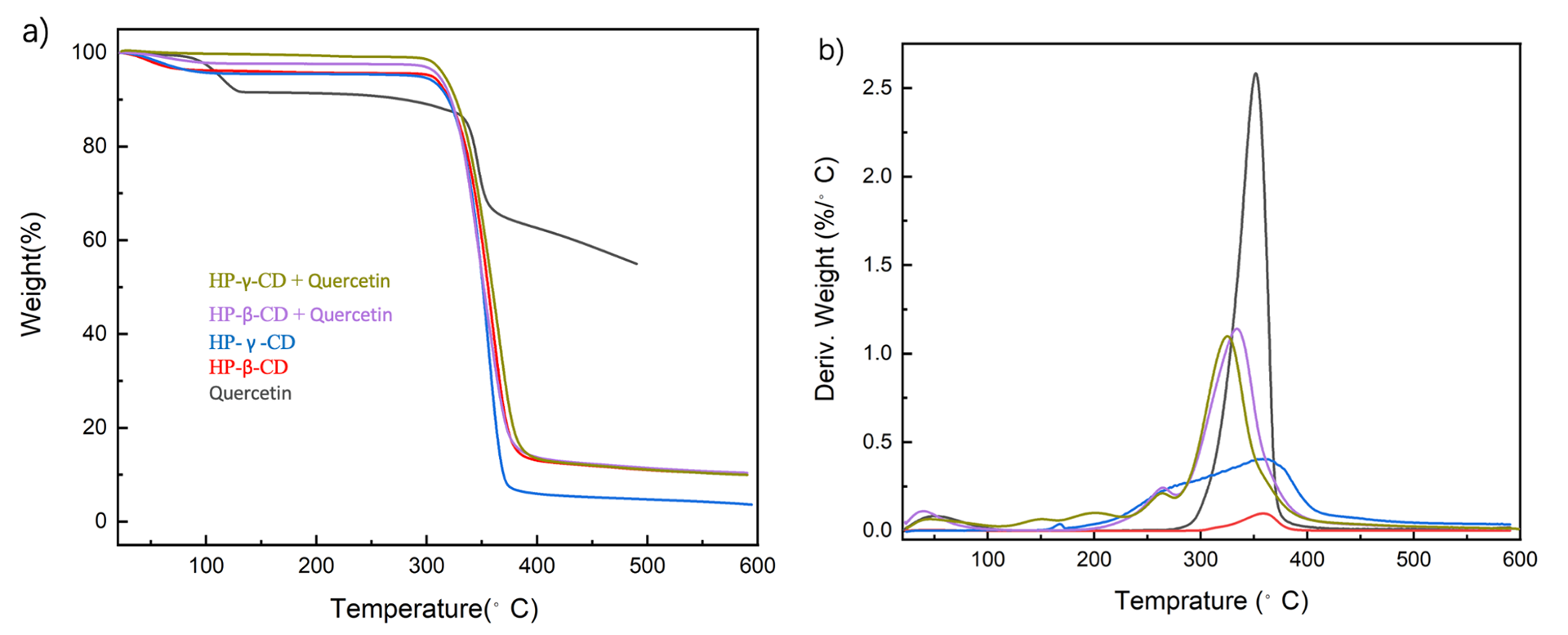
Figure 4. TGA Analysis of Quercetin + HP-β-CyD and HP-γ-CyD (Credit to Ruobai Xiao)
3.4 X-ray Diffraction
In comparison between HP-β-CyD and HP-γ-CyD nanofibrous webs, XRD was utilized instead of DSC. Sample crystallinity and structural analysis through XRD may be seen in Figure 5. Figure 5 illustrates how quercetin has characteristic peaks at 10.8°, 12.5°, 15.8°, 16.2°, 23.9°, 24.8°, 26.5°, and 27.4° that seem to align with peaks in the Qu/CyD samples tested. Within both Qu/HP-β-CyD and Qu/HP-γ-CyD samples, broader peaks further reinforce the nanocrystalline size of the nanofibers while the noisiness of the curves indicate some amorphous properties of the nanofibrous webs. Additionally, the more similar peaks of Qu/HP-γ-CyD to its corresponding CyD and quercetin peaks demonstrate higher nanofibrous web quality with greater encapsulation.
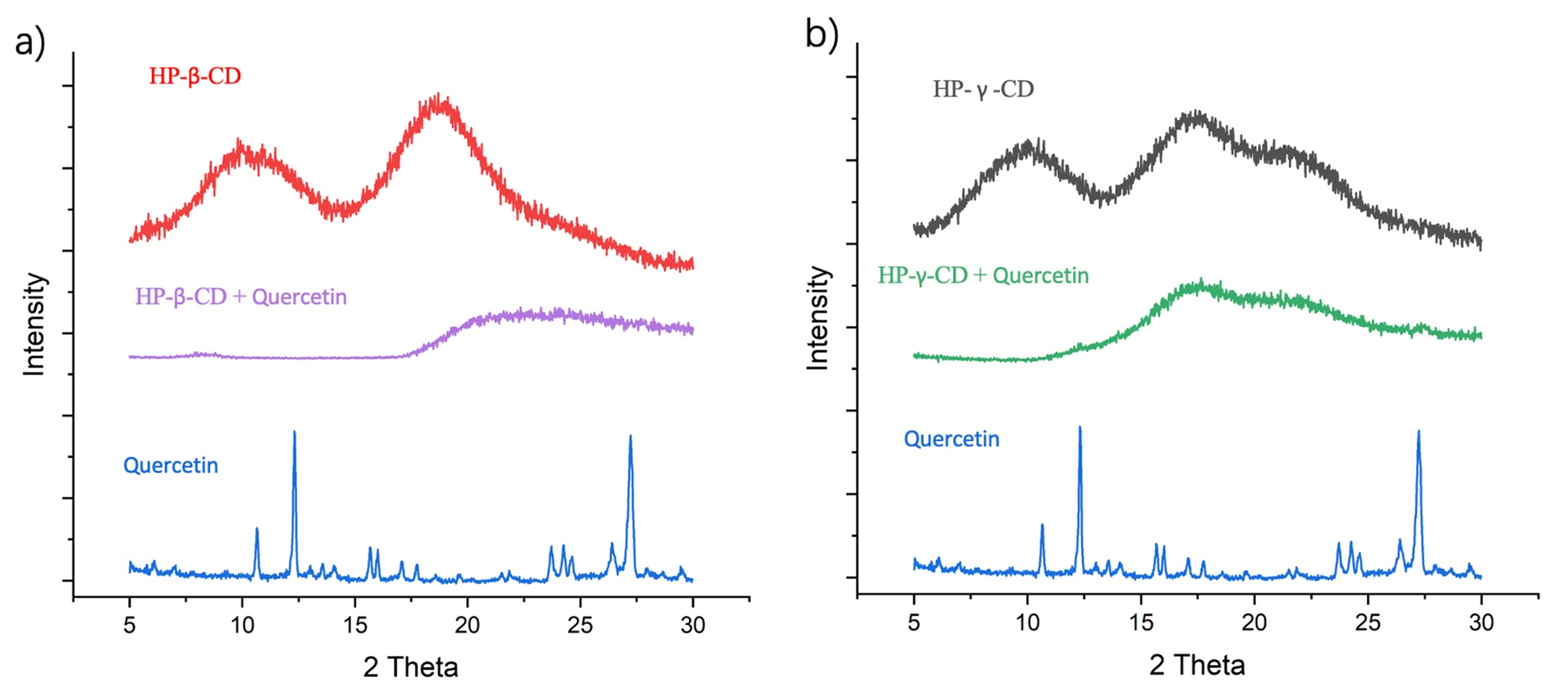
Figure 5. X-ray Diffraction (Credit to Ruobai Xiao)
3.5 Antioxidant Test
A lack of time prevented the execution of the antioxidant test on quercetin samples leading to a lack of antioxidant data. However, experimental procedure and understanding was achieved through aiding the testing of curcumin samples. Due to the similar anti-oxidative nature of curcumin and quercetin, test results and process should be highly similar.
3.6 Sustained Release Test
Similar to the antioxidant test, sustained release test was unable to be executed for quercetin samples. Instead, understanding was attained through shadowing the process for nanofibrous webs encapsulating curcumin within CyD.
Specifically, the difficulty of electrospinning samples containing crosslinking SHP and citric acid contributed to the inability to conduct the sustained release test for quercetin samples. The crosslinking should allow for a more slow, controlled release of quercetin compared to non crosslinked samples.
3.7 Phase Solubility Test
Time restraints also prevented the phase solubility test from being conducted for quercetin samples. Learning was achieved through observing the process of curcumin samples. From shadowing this process, it was determined that an increase in cyclodextrin concentration allows for a higher loading efficiency of drugs such as quercetin.
4. CONCLUSION
Successful creation of nanofibrous webs is illustrated through periodic microscopy during the electrospinning process and afterwards through SEM analysis. It was found that Qu/β-CyD (1:8) IC samples had the highest fiber quality due to its homogenous morphology and lack of beading or splashing. Viscosity and conductivity tests allowed for further analysis of fiber diameter and Qu/CyD concentration with HP-ɣ-CyD samples having thicker diameters.
Beyond further confirmation of structural composition of samples, DSC was unhelpful in comparing HP-β-CyD and HP-γ-CyD nanofibrous webs. Thus, XRD was used to compare the samples, illustrating how HP-γ-CyD was more successful in attaining higher nanofibrous web quality. Additionally, similar inflection points of each curve in TGA analysis demonstrated fairly high purity and desired composition of drug-encapsulated samples. Unfortunately, antioxidant, sustained release, and phase solubility tests were unable to be performed for quercetin samples.
References
1. Grand View Research. (2022). Wound dressing market size, share & trends report, 2030. Wound Dressing Market Size, Share & Trends Report, 2030. Retrieved December 13, 2022, from https://www.grandviewresearch.com/industry-analysis/wound-dressing-market
2. Jiajia Xue, Tong Wu, Yunqian Dai, and Younan Xia. (2019). Electrospinning and Electrospun Nanofibers: Methods, Materials, and Applications. Chemical Reviews 2019 119 (8), 5298-5415 DOI: 10.1021/acs.chemrev.8b00593
3. Alexander David, Radhakrishnan Arulmoli, and Subramani Parasuraman. (2016). Overviews of Biological Importance of Quercetin: A Bioactive Flavonoid. National Library of Medicine. DOI: 10.4103/0973-7847.194044
4. Asli Celebioglu and Tamer Uyar. (2020). Fast-dissolving antioxidant curcumin/cyclodextrin inclusion complex T electrospun nanofibrous webs. Elsevier. https://doi.org/10.1016/j.foodchem.2020.126397